"BRAIN MAPPING - DECODING THE HUMAN BRAIN'S COMPLEX CIRCUITRY": DE MODE GLOBAL
- DE MODE
- Apr 5, 2024
- 4 min read
ORIGINALLY PUBLISHED IN DE MODE | SCIENCE & TECHNOLOGY
Article Published on: 05TH APR 2024 | www.demodemagazine.com
Brain mapping, a multifaceted endeavor at the intersection of neuroscience, technology, and computational sciences, embodies the relentless pursuit of understanding the intricate circuitry of the human brain. This ambitious pursuit aims to decipher the enigmatic complexities of the brain's structure and function, unlocking profound insights into cognition, behavior, and neurological disorders. In this exploration, we delve into the fascinating world of brain mapping, unveiling its methodologies, applications, challenges, and transformative potential.
At the heart of brain mapping lies the quest to unravel the brain's complex network of neurons, synapses, and neurotransmitters, which underlie every aspect of human experience. This intricate circuitry orchestrates a symphony of electrical and chemical signals, giving rise to consciousness, memory, emotions, and every facet of cognition. By mapping this neural landscape, scientists endeavor to elucidate the fundamental principles governing brain function, akin to deciphering the code of a biological supercomputer.

Advances in neuroimaging techniques have revolutionized the field of brain mapping, enabling researchers to peer into the inner workings of the brain with unprecedented precision and resolution. Techniques such as functional magnetic resonance imaging (fMRI), electroencephalography (EEG), magnetoencephalography (MEG), and positron emission tomography (PET) allow for non-invasive probing of brain activity, unveiling the dynamic interplay of neural circuits during various cognitive tasks and states. These imaging modalities provide a window into the brain's functional architecture, revealing regions specialized for sensory processing, motor control, language, emotion regulation, and higher-order cognition.
In tandem with functional imaging, structural imaging techniques such as diffusion tensor imaging (DTI) and magnetic resonance spectroscopy (MRS) offer insights into the brain's anatomical organization and chemical composition. DTI, for instance, maps the diffusion of water molecules along white matter tracts, delineating the brain's intricate connectivity patterns. MRS, on the other hand, detects concentrations of various neurotransmitters and metabolites, shedding light on biochemical processes underlying brain function and dysfunction.
Beyond imaging, neuroscientists harness the power of electrophysiology to probe the brain's electrical activity at the cellular and network levels. Techniques such as single-unit recording, patch clamping, and multi-electrode arrays enable precise monitoring and manipulation of neuronal activity, unraveling the dynamics of neural circuits with unparalleled temporal resolution. These approaches offer crucial insights into the spatiotemporal patterns of neural activity underlying perception, learning, memory, and other cognitive processes.
In recent years, the advent of optogenetics has revolutionized the field of brain mapping, empowering researchers to selectively activate or inhibit specific populations of neurons with light. By genetically engineering neurons to express light-sensitive proteins, scientists can precisely control neural activity with millisecond precision, unraveling the causal relationships between neuronal dynamics and behavior. Optogenetics holds immense promise for dissecting the neural circuits underlying complex phenomena such as decision-making, addiction, and psychiatric disorders.
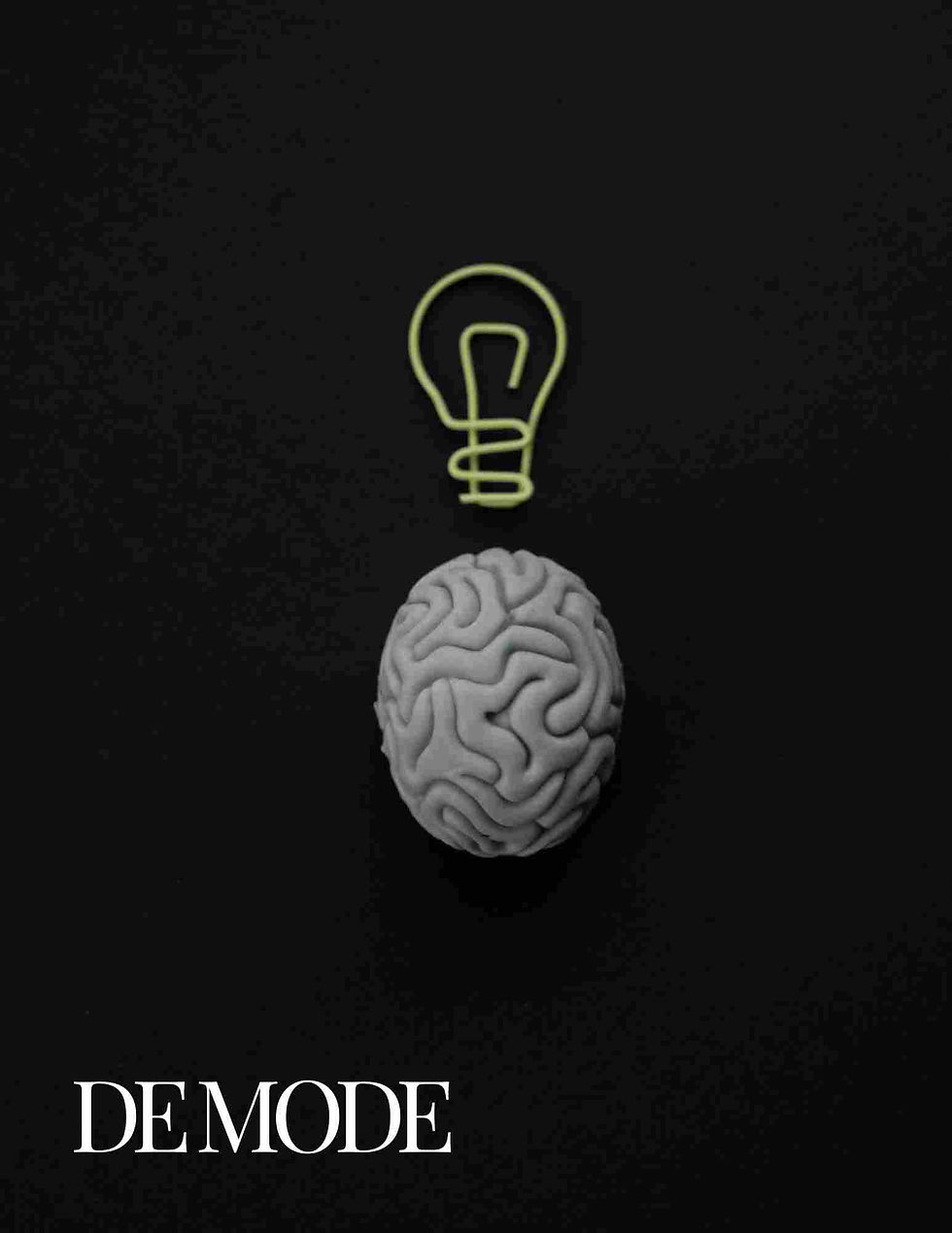
Complementing experimental approaches, computational modeling plays a pivotal role in brain mapping, facilitating the synthesis of vast experimental data into coherent theoretical frameworks. Computational models simulate the behavior of neural circuits, elucidating how patterns of activity emerge from the interactions of individual neurons and synapses. These models range from simplified spiking neuron models to biophysically detailed simulations of entire brain regions, providing valuable insights into the mechanisms underlying brain function and dysfunction.
The applications of brain mapping are as diverse as they are profound, spanning basic research, clinical neuroscience, and technology development. In basic research, brain mapping sheds light on the neural mechanisms underlying perception, cognition, and behavior, advancing our understanding of the mind-brain relationship. In clinical neuroscience, brain mapping holds promise for diagnosing and treating neurological and psychiatric disorders, ranging from Alzheimer's disease and Parkinson's disease to depression and schizophrenia. By pinpointing aberrant patterns of brain activity, clinicians can develop targeted interventions such as deep brain stimulation, transcranial magnetic stimulation, and pharmacotherapy, tailored to individual patients' neural profiles.
Moreover, brain mapping fuels the development of brain-computer interfaces (BCIs), neuroprosthetics, and brain-inspired AI systems, blurring the boundaries between man and machine. BCIs translate neural activity into control signals for external devices, enabling paralyzed individuals to communicate, move prosthetic limbs, and even restore sensory feedback. Neuroprosthetics, such as cochlear implants and retinal implants, bypass damaged sensory organs, directly stimulating the brain to restore lost sensory functions. Meanwhile, brain-inspired AI systems draw inspiration from the brain's architecture and computational principles, paving the way for next-generation AI algorithms capable of learning, reasoning, and adapting in complex environments.
Despite its transformative potential, brain mapping faces formidable challenges on multiple fronts. The sheer complexity of the brain's circuitry, with its billions of neurons and trillions of synapses, poses a daunting obstacle to comprehensive mapping efforts. Moreover, the brain exhibits remarkable plasticity, constantly reshaping its connectivity in response to experience, development, and pathology, further complicating mapping endeavors. Technical limitations, such as spatial and temporal resolution constraints, noise, and artifacts, impose further constraints on imaging and recording techniques, necessitating continuous innovation and refinement.
Ethical considerations also loom large in the pursuit of brain mapping, particularly concerning privacy, consent, and the responsible use of neurotechnologies. The ability to decode thoughts, emotions, and intentions from neural activity raises profound ethical dilemmas regarding personal autonomy, mental privacy, and the potential for misuse or abuse. Moreover, the unequal distribution of neuroscientific resources and expertise raises concerns about exacerbating existing disparities in healthcare, education, and access to neurotechnologies.

In conclusion, brain mapping stands at the forefront of scientific inquiry, poised to unlock the mysteries of the human brain and revolutionize our understanding of the mind. By combining cutting-edge imaging technologies, experimental techniques, computational modeling, and interdisciplinary collaboration, brain mappers strive to decipher the brain's complex circuitry, illuminating the pathways from neurons to consciousness. As we unravel the brain's secrets, we pave the way for transformative advances in neuroscience, medicine, technology, and beyond, ushering in a new era of understanding and enhancing the most complex organ in the known universe: the human brain.
Comments